Through the Looking Glass: The Evolution of Microscopy

Imaging biomolecules, microorganisms, and viruses not perceivable by the naked eye has always been challenging for biologists. Ever since Robert Hooke’s visualization of dead cork cells in 1665 and Anton van Leeuwenhoek’s observation of bacteria using a self-made single-lens microscope in 1674, the use of microscopy in biology has come a long way. With the fairly recent development of cryo-electron microscopy that fetched the 2017 Nobel Prize in Chemistry (awarded to Jacques Dubochet, Joachim Frank, and Richard Henderson), microscopy continues to revolutionize the field of structural biology and influence the way we understand biomolecules and living systems.
(Image of Richard Henderson, Joachim Frank, and Jacques Dubochet)
Practically speaking, microscopy has long since surpassed its ability to allow scientists to simply “see” cells and molecules. Microscopes have played a crucial role in the development of important fields such as cytogenetics (pioneered by Walther Flemming after his observation of mitosis in 1882), microscopic anatomy (Marcello Malpighi after his observation of red blood cells in 1666), as well as the discovery of the nucleus (Robert Brown in 1831) and ribosomes (George Palade in 1955). Today, the use of fluorescent proteins as reporter molecules has allowed the visualization and live tracking of biomolecules and helped us understand the intracellular dynamics like never before.
Major Highlights in History
The 19th and 20th century saw a rapid evolution of microscopes from an elementary combination of lenses for magnification (Joseph Jackson Lister in 1826) to complicated systems employing electrons to enhance resolution (Ernst Ruska in 1933).
In the late 1590s, Hans Lippershey and his son, Zaccharias Janssen, serendipitously invented the compound microscope when they arranged several lenses in a tube and observed that the object placed in front of it could be magnified to a much larger extent than a magnifying glass. Later in the 17th century, Hooke and Leeuwenhoek paved the way for development of cell theory by their first-ever observation of cells.
In the 1730s, Chester Moore Hall invented the achromatic lens using which he showed that a second lens with different refractive properties could be used to realign colors without disrupting magnification by the first one, thereby eliminating chromatic aberration. Then in 1830, Joseph Jackson Lister solved the problem of spherical aberration by placing lenses at appropriate distances from each other. Following the development of the Abbe condenser by Ernst Abbe in 1870 (that condenses and controls the light entering the specimen), further developments in microscopy were primarily based on a systematic study of the laws of physics, instead of the trial-and-error method that had been followed until then.
Light microscopy
(Image of light microscope lens - stock photo)
Perhaps the first light microscope ever used was by Hooke to observe the cork cells (he used a candle to illuminate his specimen under a single lens microscope). The most basic form of an optical microscope is the bright-field microscope that illuminates the specimen with the light that passes through it, forming the image. Another system is the dark-field microscope that lights the specimen from an angle and only scattered rays form the image. Multiple modifications have also been made in light microscopes to improve imaging such as:
- optimization of contrast (phase-contrast microscope/differential interference contrast microscope)
- use of digital techniques to enhance image processing [charge coupled devices (CCDs) or high sensitivity complementary metal-oxide-semiconductor (CMOS) sensors]
- use of fluorescent dyes and antibodies (immunocytochemistry) to enhance staining (fluorescence microscope)
- generating 3D images by focusing on one plane of specimen at a time and then stacking the planes together (confocal microscope)
The first Nobel Prize for microscopy was awarded in Physics to Frits Zernike in 1953 for the invention of the phase-contrast microscope.
UV microscopy
By the start of the 20th century, it had become known that visible light was the limiting factor in the extent of resolution obtained. Thus, the limit of resolution could be defined by the wavelength of radiation used - smaller the wavelength, better the resolution. This prompted Zeiss (a German manufacturer of optical systems and optoelectronics, founded in 1846 by optician Carl Zeiss) to employ UV as the radiation source (given the comparatively lower wavelength of the UV range) and thus, the first commercial UV microscope was introduced in 1904 (developed by August Köhler and Moritz von Rohr).
Electron microscopy
When the dual nature of electrons was established, scientists turned their attention to the possibility of using accelerated electrons as a source of illumination. Since the wavelength of electrons is much lesser than visible light (plus the difficulty in correcting aberrations in electron lenses), the effective resolution of an electron microscope is 1 nm - around 200 times better than that of a light microscope! Thus, in 1931, the first electron microscope was invented by Ernst Ruska (and Max Knoll) that later won him the Nobel Prize in Physics in 1986.
(Image of SEM vs TEM)
The scanning and the transmission electron microscopes are the two main types of electron microscopes. The major difference between the two lies in the way the electrons illuminate the sample and produce an image. SEM creates an image by detecting electrons scattered by the specimen while TEM detects electrons transmitted through the specimen. Thus, SEM can generate 3D images primarily of the surfaces of the specimen while TEM gives a 2D image of its internal composition.
What followed later was an overwhelming upsurge in the determination of structures of biomolecules like proteins, viruses, and macromolecular cellular machinery. Nuclear Magnetic Resonance (NMR) Spectroscopy, X-ray crystallography, and electron microscopy have been essential players in redefining the way we understand proteins and their interactions with the cellular environment. NMR has been used to determine the atomic structure of macromolecules in solution. X-ray crystallography has been extensively used in biology for decoding the structure of proteins, nucleic acids (including the celebrated discovery of DNA double helix by Francis Crick, James Watson, and Maurice Wilkins - Nobel Prize in Physiology or Medicine 1962), and ribosomes (Venkatraman Ramakrishnan, Thomas Steitz, and Ada Yonath - Nobel Prize in Chemistry 2009). The major limitation of X-ray crystallography is that the image of the specimen is obtained in one static state. This means that the image may no longer be a faithful replica of the native conditions within the cell, in which case it would be much more dynamic and may even exist in more than one state. The second difficulty is with imaging proteins that cannot be crystallized, such as intrinsically disordered proteins. This initiated the need for the development of a system that can essentially capture the cell in its native state by minimising the perturbations caused during sample preparation, illumination, and visualization. This is where the most recent form of microscopy comes into the picture - cryo-electron microscopy.
(Image of the increasing level of resolution)
Cryo-electron microscopy
Ever since its development, cryoEM has been a promising candidate in imaging the near-native state of cells, reconstruction of 3D structure of proteins, the structure of viruses (such as the Zika virus in 2016) and shapes of biomolecules. Such structures have played important roles in understanding how proteins fold and interact with other molecules, how their defective form can lead to disease, and how they can be potential drug targets.
(image of cryo-electron microscope at MRC)
On 5th February 2020, the Electron Microscopy Data Bank (EMDB), a database of protein and other molecular structures obtained by electron microscope, celebrated the acceptance of its 10,000th entry. From 2002, there has been a linear increase in the number of entries of structures solved by X-ray crystallography, however, around 2013 onwards, there has been an exponential increase in the numbers of cryoEM entries. As of today, the cryoEM technique boasts resolution of features less than an incredible 0.2 nm in diameter. (Credit : https://www.nature.com/articles/d41586-020-00341-9)
Even though it seems that cryoEM may soon put X-ray crystallography out of business (in the words of the Nobel Laureate V. Ramakrishnan - “Nobody in my lab wanted to set up crystallization trays anymore and pray our proteins would crystallize”), it is still a long road ahead. For now and the near future, X-ray crystallography remains a standard technique of imaging proteins that can be crystallized. However, we may certainly expect to see a rapidly growing application of cryoEM in the field of structural biology as funding agencies spend tens of millions of dollars to develop cryoEM centres for greater accessibility to structural biologists around the world.
Note : Other Nobel Prizes that have been awarded for microscopy include -
- The Nobel Prize in Chemistry 2014 (Eric Betzig, Stefan W. Hell and William E. Moerner) “for the development of super-resolved fluorescence microscopy”
- The Nobel Prize in Physics 1986 (Ernst Ruska) “for his fundamental work in electron optics, and for the design of the first electron microscope” and (Gerd Binnig and Heinrich Rohrer) “for their design of the scanning tunneling microscope”
- The Nobel Prize in Chemistry 1982 (Aaron Klug) “for his development of crystallographic electron microscopy and his structural elucidation of biologically important nucleic acid-protein complexes”.
Author Bio: Minita Desai graduated from IISER Bhopal's BS-MS programme in 2021, majoring in Biological Sciences. She carried out her Master's thesis research in the Chaperone and Stress Biology Laboratory, as a part of Dr. Chandan Sahi’s lab group. An INSPIRE scholar, her principal research interest lies in investigating cell regulation on a molecular scale. Be it chaperones in yeast or ribosomes in bacteria, narrowing down who flips the switches and regulates the insides of a cell is what unfailingly piques her curiosity. Desirous of enjoying more congenial weather after tolerating five years of Bhopal's wild swings, she is looking forward to pursue her doctoral studies at NCBS/InStem in Bangalore.
Further Reading:
- A cold, clear view of life wins chemistry Nobel: https://www.sciencemag.org/news/2017/10/cold-clear-view-life-wins-chemistry-nobel
- Revolutionary cryo-EM is taking over structural biology : https://www.nature.com/articles/d41586-020-00341-9
- With cryo-electron microscope, India hopes to join the revolution sweeping across the world of medicine : https://economictimes.indiatimes.com/news/science/with-an-expensive-microscope-india-hopes-to-join-the-revolution-sweeping-across-the-world-of-medicine/articleshow/62865148.cms
- ‘We need a people’s cryo-EM.’ Scientists hope to bring revolutionary microscope to the masses : https://www.sciencemag.org/news/2020/01/we-need-people-s-cryo-em-scientists-hope-bring-revolutionary-microscope-masses
5. Scientific Background on the Nobel Prize in Chemistry 2017 (The Development of cryo-electron microscopy) : https://www.nobelprize.org/uploads/2018/06/advanced-chemistryprize2017.pdf
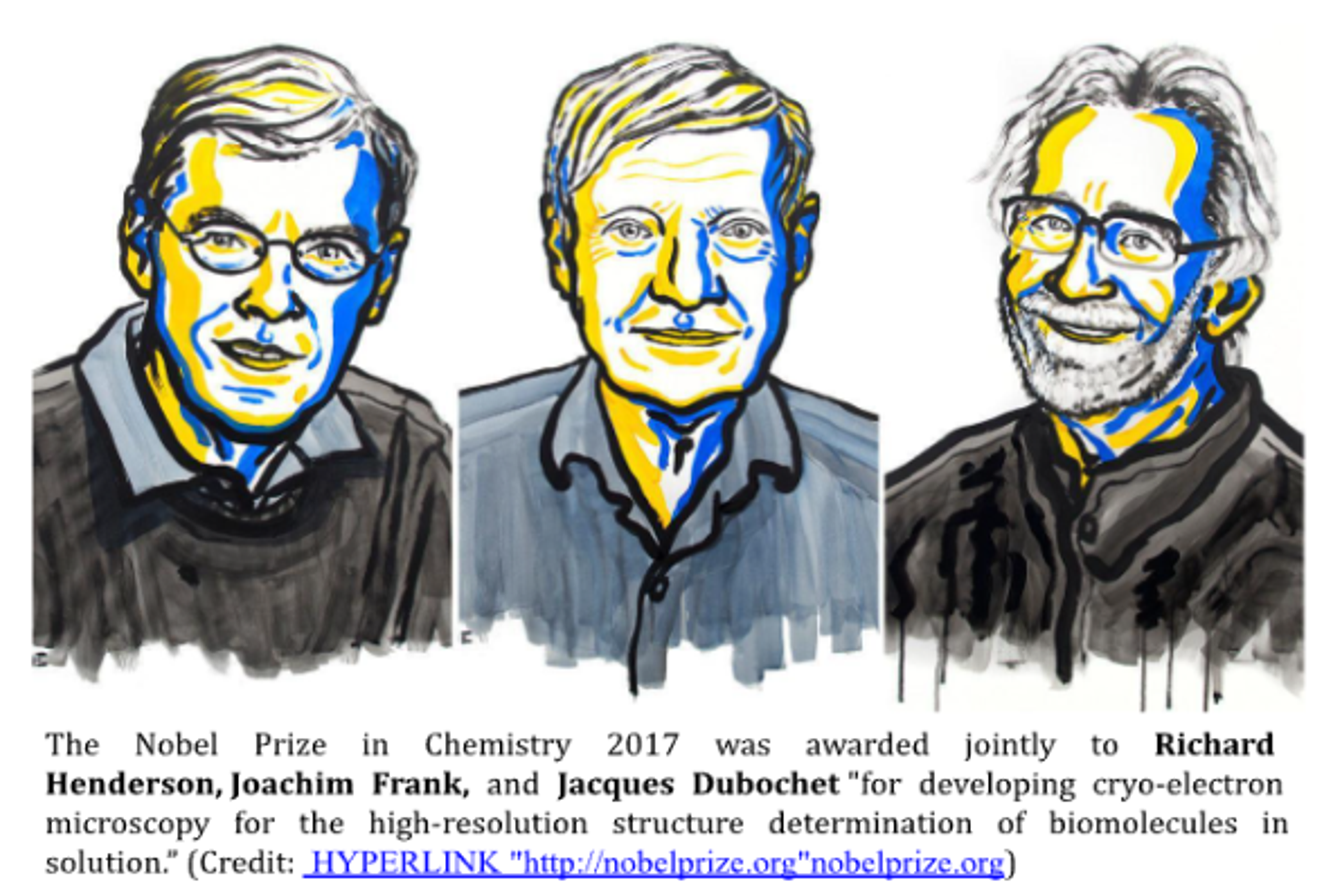
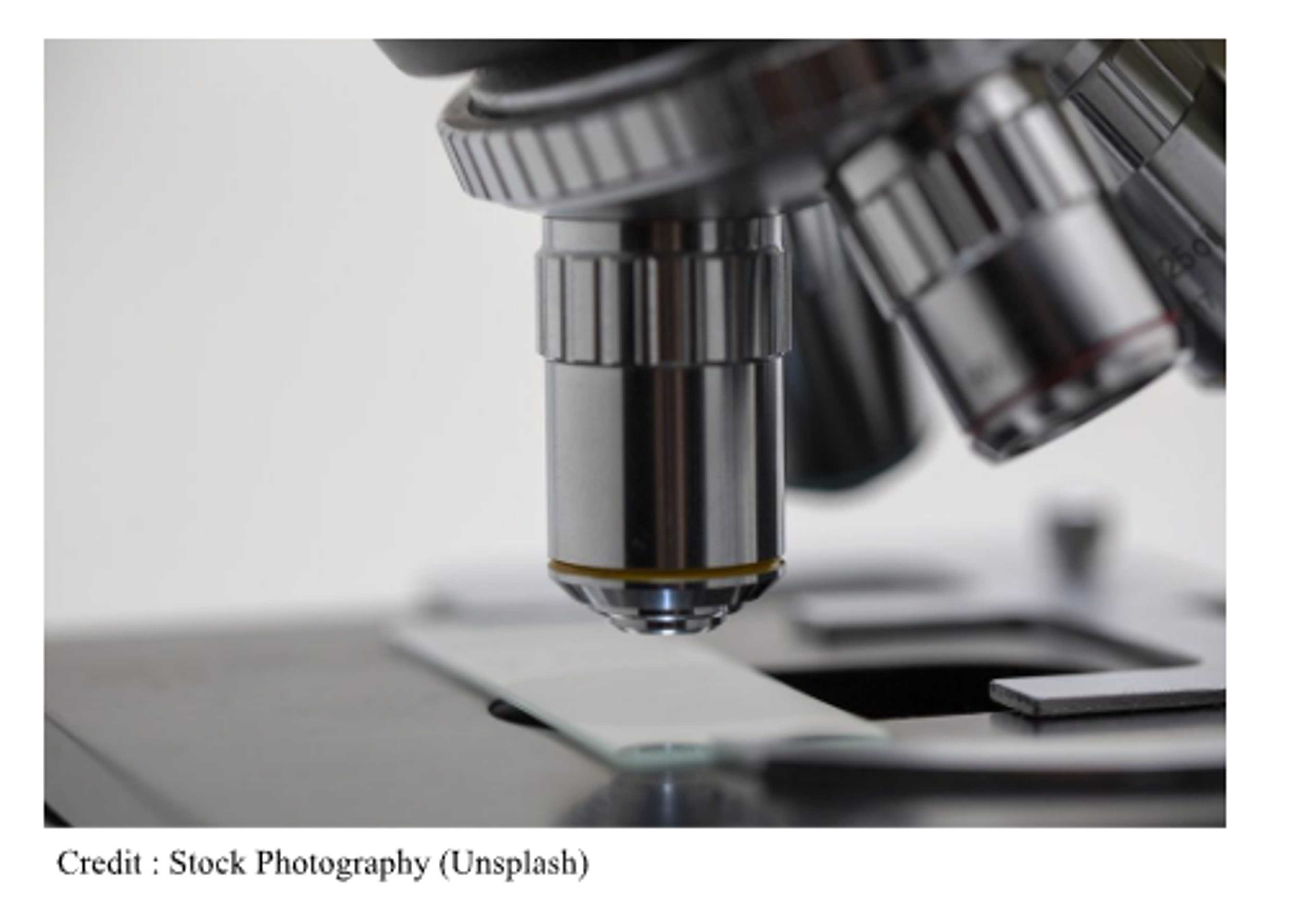
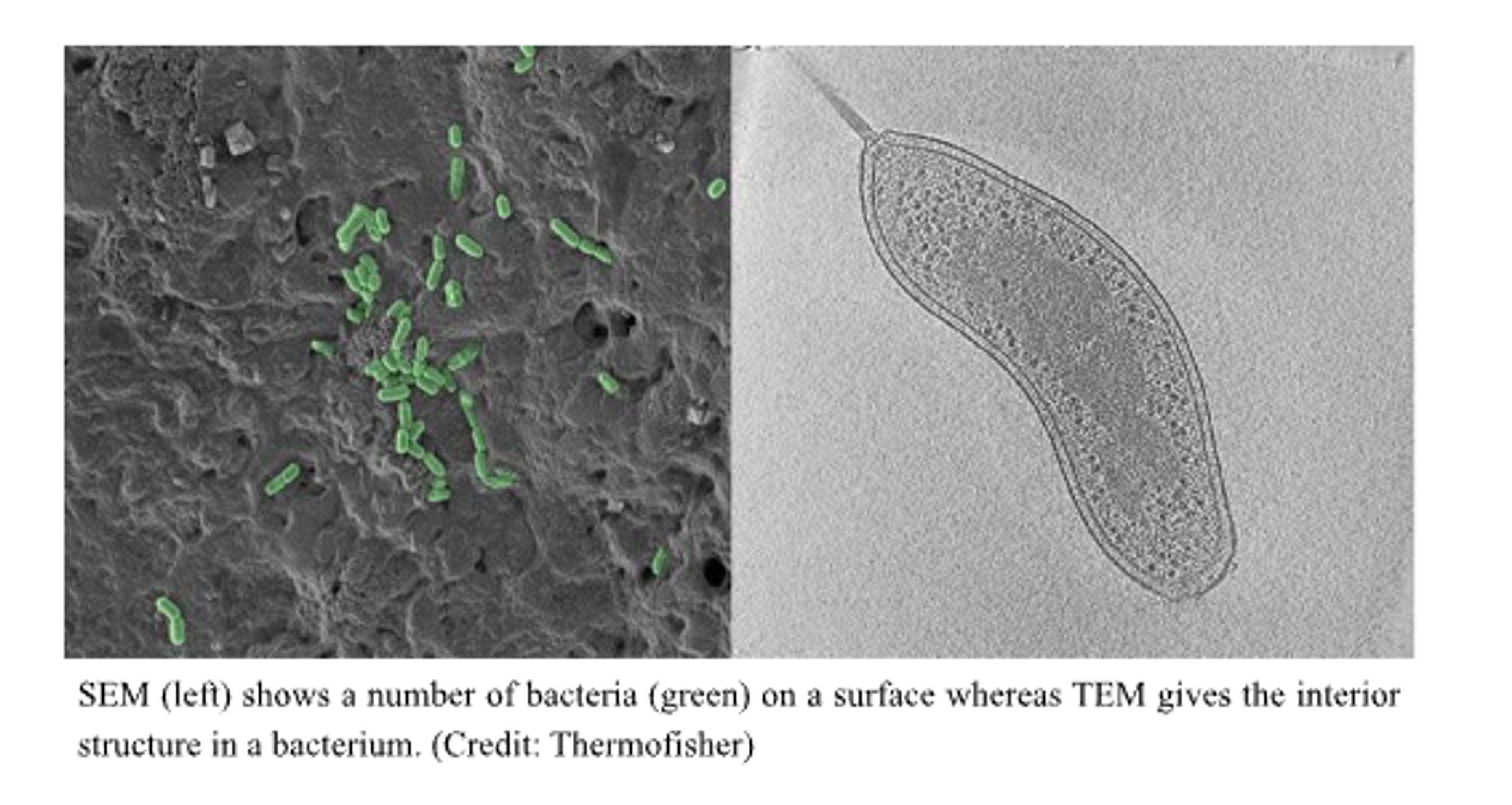
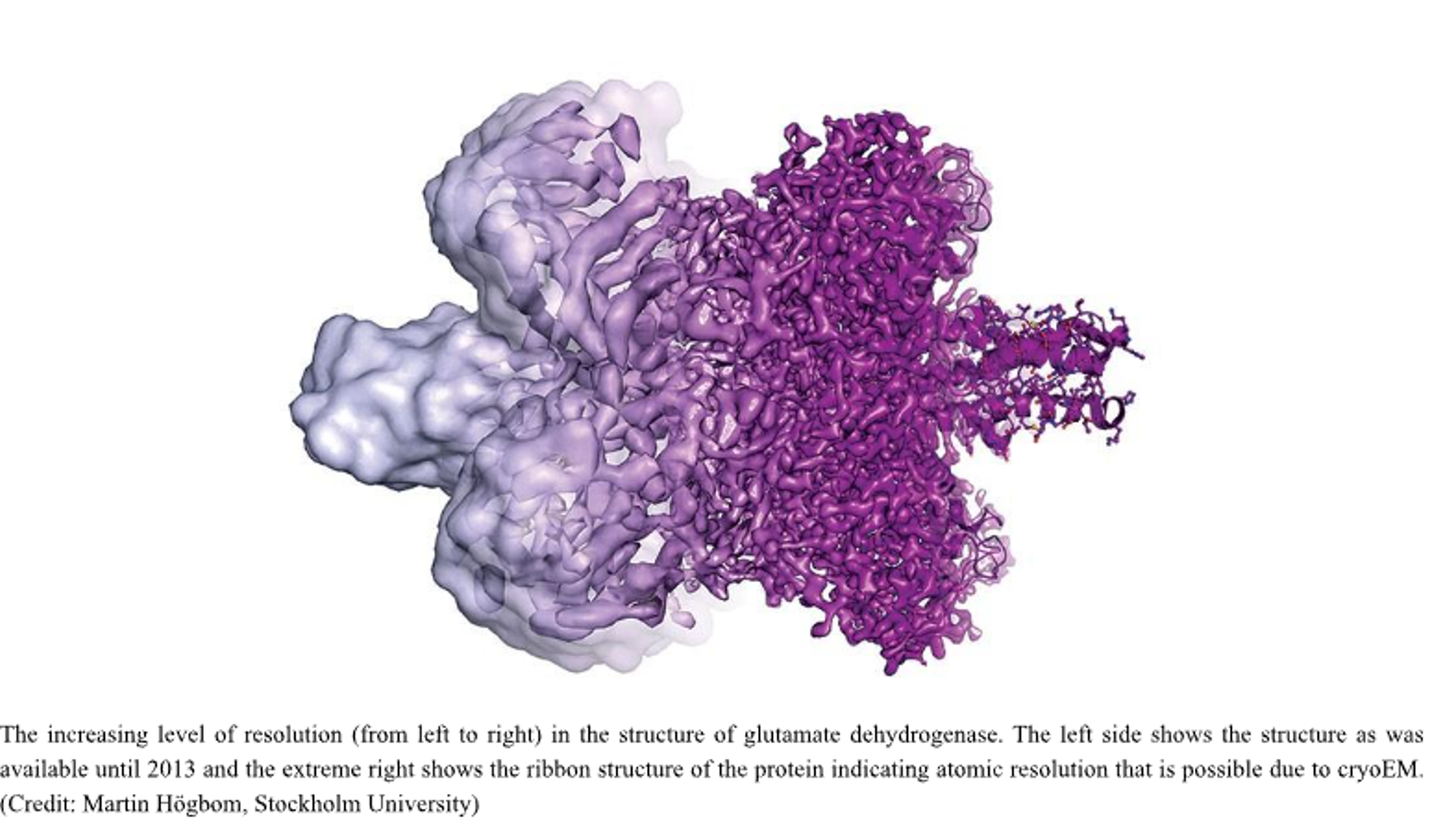
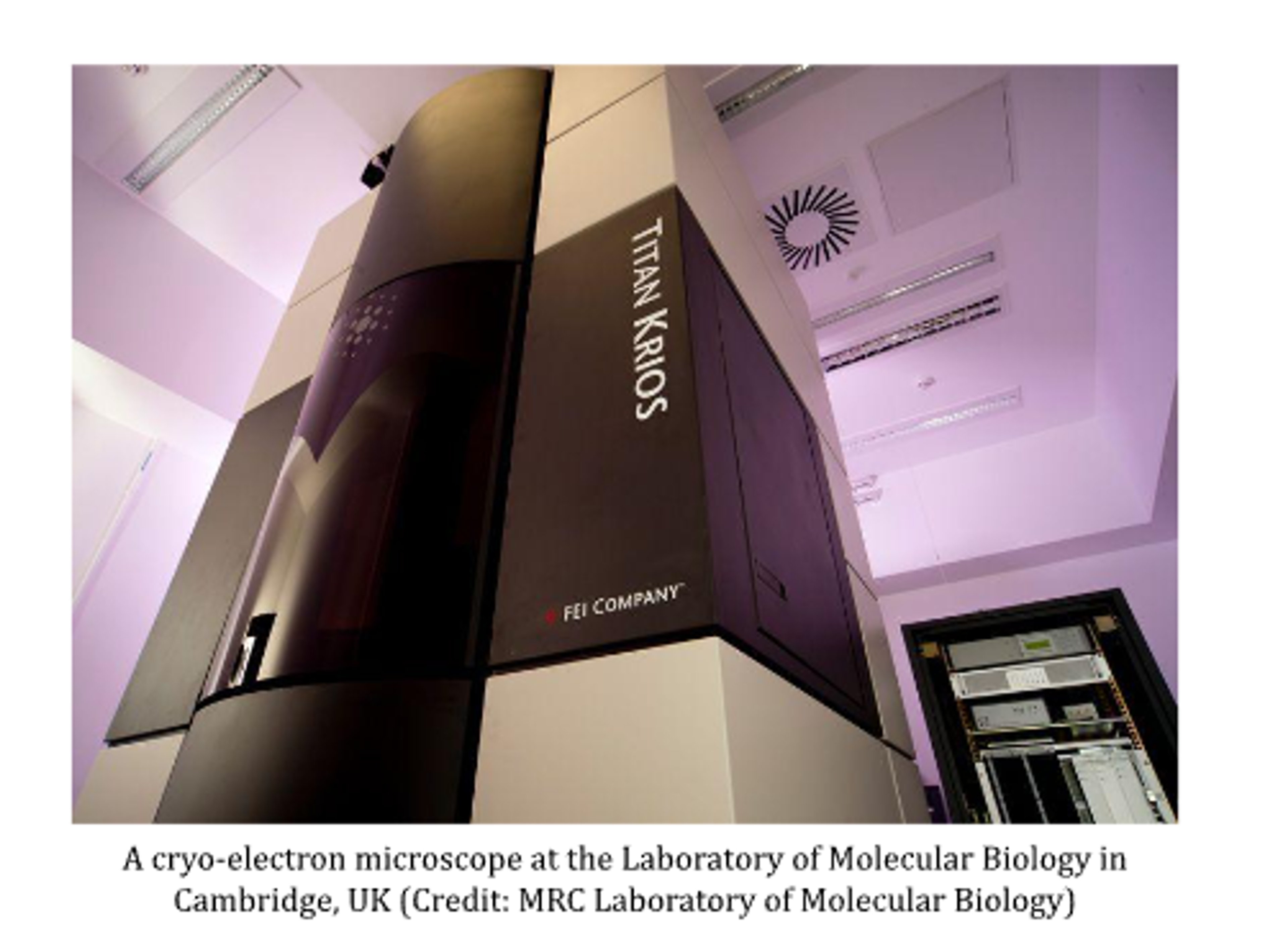