Earth as a Complex System
"There's no love in a carbon atom, No hurricane in a water molecule, No financial collapse in a dollar bill." – Peter Dodds
Firstly, what is a complex system?
Complex systems science investigates how a diverse set of locally coupled components can self-organize to demonstrate non-trivial patterns (emergent behavior) at larger scales. This emergent behavior refers to collective behavior in complex systems that are not present in their individual components. Thus, the properties of the collection may not be understood by examining its specific components alone. Such collection of nonlinearly interacting components is called a complex system, and its examination necessitates the development of new mathematical frameworks and scientific methodologies. Examples of complex systems include the human brain, cities, social organizations, ecosystems, climate systems, and, eventually, the entire universe.
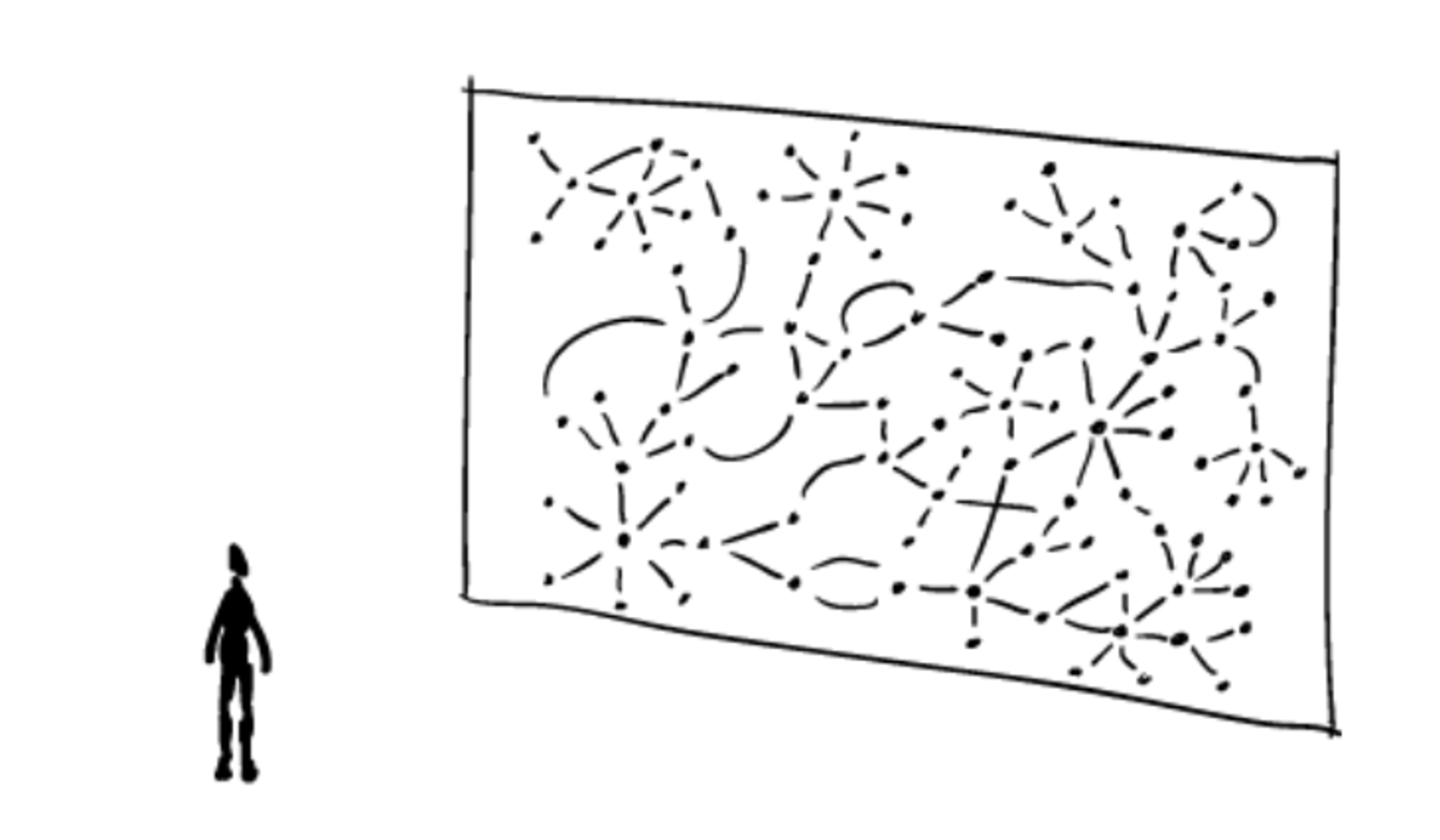
Earth systems are extremely dynamic, with multiple nonlinear subsystems. The first step in conceptualizing the earth as a complex system is to narrow down our focus and study the emergence of specific sub-systems (studying the earth as one complex system is ideal but practically not possible). Earth’s subsystems such as climate, ecosystems, and river systems can be studied as individual complex systems. For instance, the earth’s climate can be modelled as a complex system with spatiotemporal variation in wind, temperature and precipitation resulting in the emergence of cyclones, floods, and droughts. Earth's ecosystems are another example of complex systems, with their emergence dependent on the presence of various biotic and abiotic components, as well as the associated eco-geomorphic processes and climatic perturbations. Eco-geomorphological concepts are attributed to the fact that animals and plants inhabiting an ecosystem can effectively modulate the flows of energy and matter, characterizing the biogeochemical cycles, sediment transport, and emergence of unique landscapes. At the same time, surrounding geomorphic processes can also affect the survival of the organism. For example, Wainwright et al. (2002)1 exhibited that depository beads along small channels in New Mexico were the focus of increased vegetation growth. In addition, Reinhardt et al (2010)2 advocated that life and its landscape each exerts a simultaneous influence on the other over a wide range of temporal and spatial scales.
Another important characteristic of the Earth’s ecosystems (complex systems) is connectivity, which is defined as the level of connectedness within a system (Turnbull et al., 2008)3. Connectivity can be further subdivided into structural and functional templates. For example, in river systems, structural connectivity is the channel network (driven by topography), whereas functional connectivity is the movement of water, sediments, and riverine species along with the structural template.
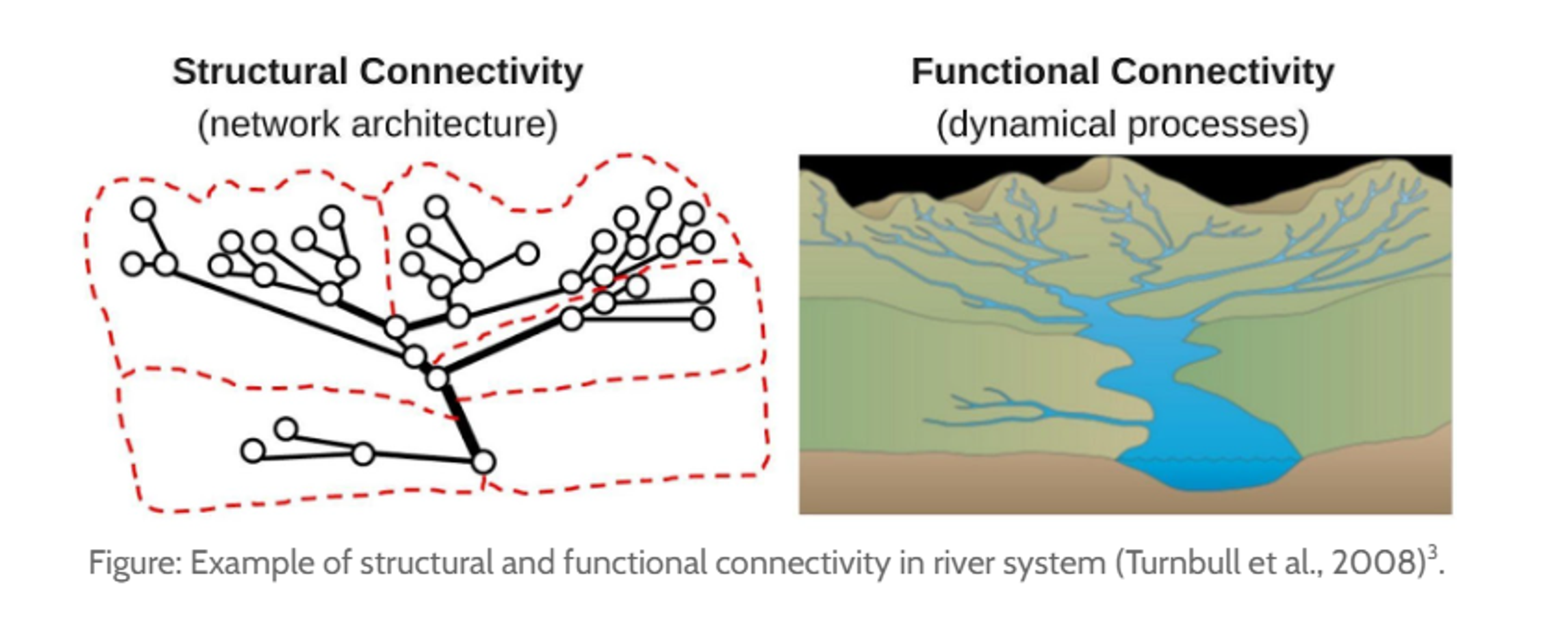
Figure: Example of structural and functional connectivity in river system (Turnbull et al., 2008)3.
In recent years, network theory has been used to quantify connectivity in complex systems, where a network is a graph-based representation of a system with nodes and links. (see Barabási (2012) ^4, for a detailed description of Network Theory). The key idea behind network science is that it is possible to obtain crucial information about a complex system by studying its underlying network structure. Ecosystems can be represented as a multilayer network because of the presence of climatic (precipitation, temperature, and wind speed), biotic (plant cover and animals), and abiotic (topography and soil type) components.
The multilayer network representation of an ecosystem incorporates layers of structural connectivity, functional connectivity, and climate forcing. The characteristics of inter and intra-layer connections in the resulting network allow us to understand the emergence of an ecosystem. For instance, the structural connectivity network associated with an eco-geomorphic system can be constructed based on the topography-based flow routing (movement of water and sediments from high to low elevation nodes) and presence of vegetation sinks (vegetation cover act as sink to trap water and sediment). Furthermore, the functional connectivity network depends on the magnitude of fluxes of water and sediment along the structural template. (Turnbull and Wainwright, 2019) ^5 . The nodes in this case are vegetation patches, and the links are formed by the water and nutrient
fluxes between them.
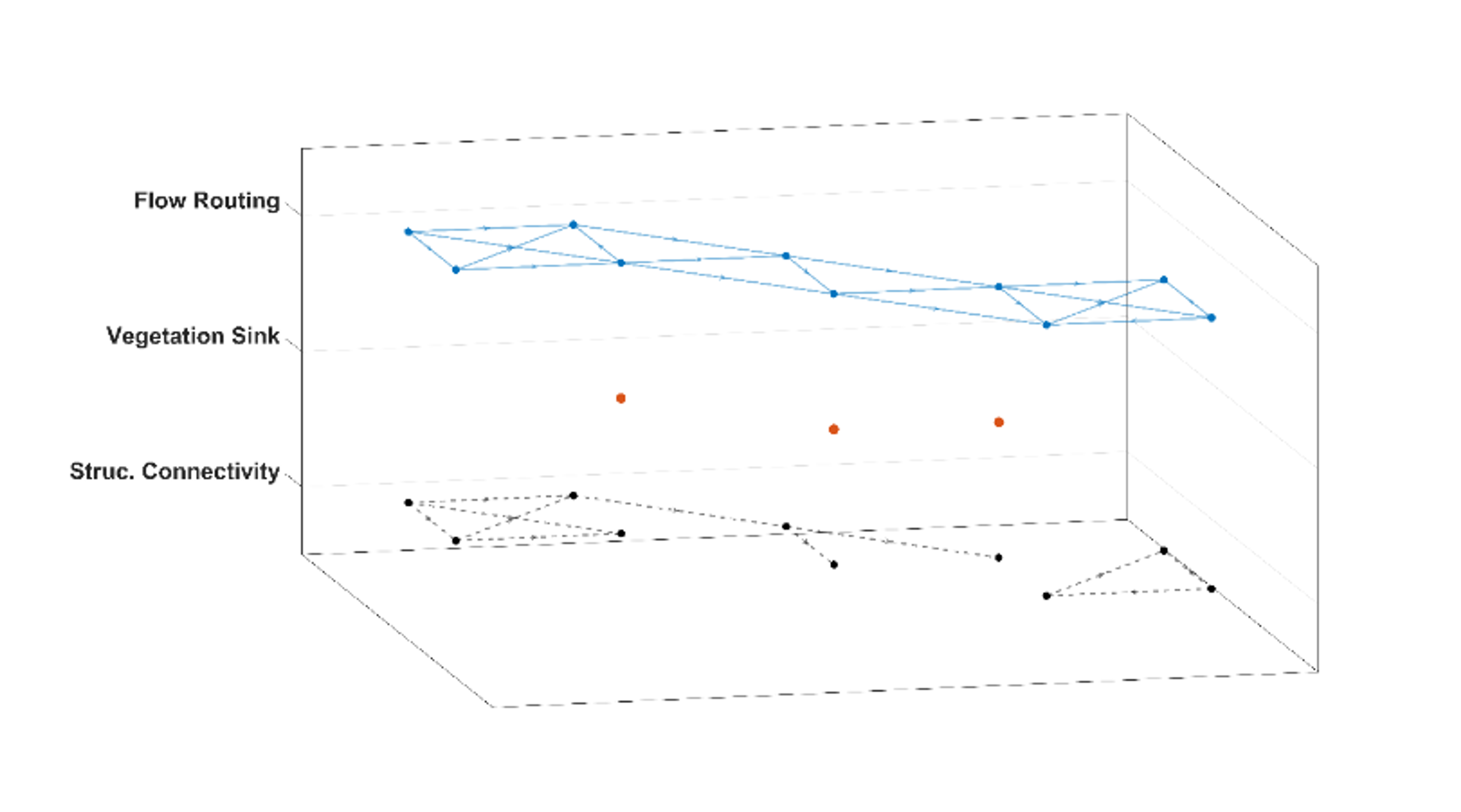
Figure: The conception of a structural connectivity network based on flow routing and the presence of vegetation sinks.
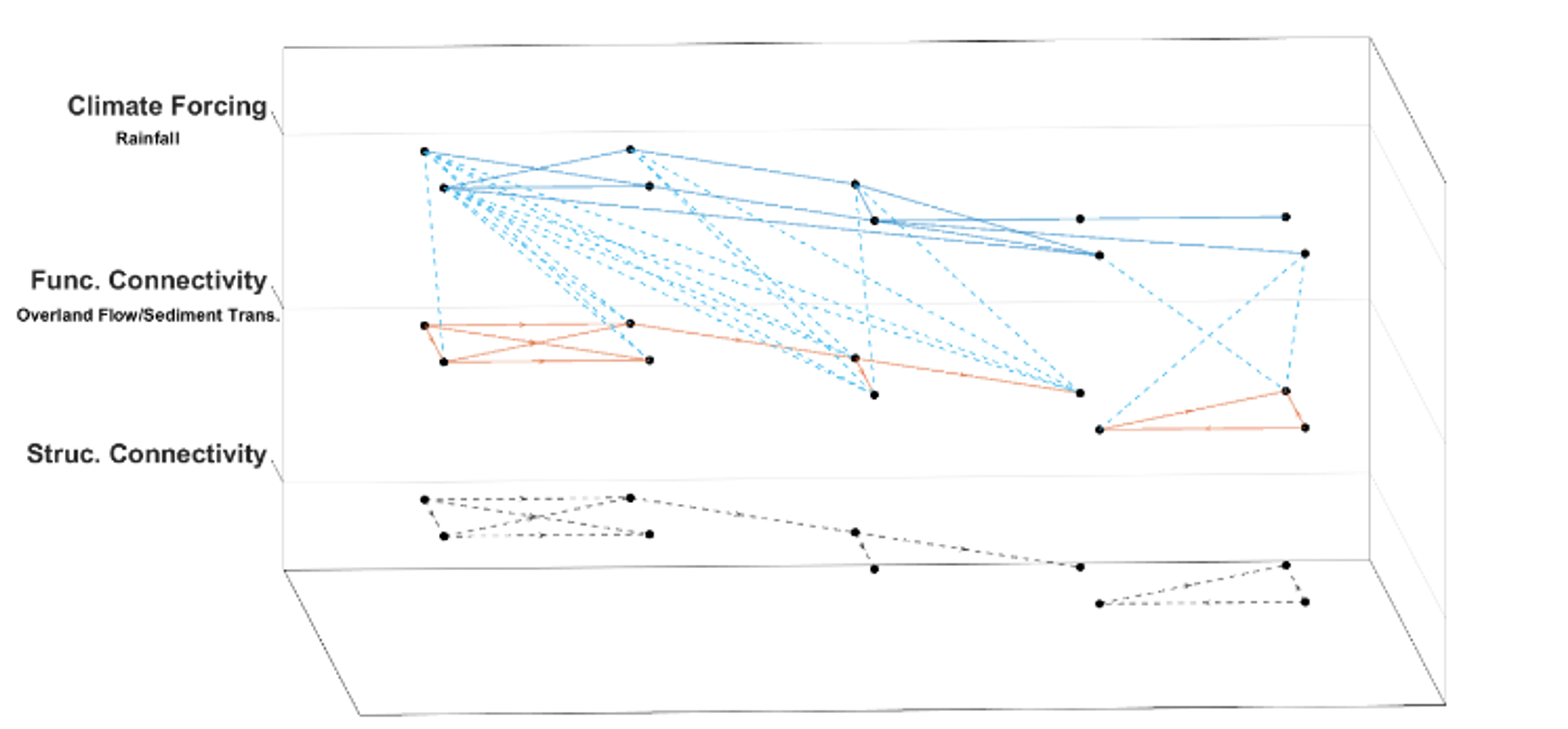
Figure: The representation of an eco-geomorphic system as a multilayer network.
These are just initial conceptualizations of the network-based representation of an ecosystem.The proposed network-based approach will enable us to identify critical
nodes in these systems, allowing us to develop appropriate management strategies to
preserve the current state of the ecosystem. I am currently working as a Ph.D. student under the supervision of Dr. Laura Turnbull-Llyod and Prof. John Wainwright to better understand the emergence of dryland ecosystems, particularly concerning land degradation-related processes.
- Further information about my Ph.D. project: Shubham-Tiwari | Durham University
- Further reading: Turnbull, L., Hütt, MT., Ioannides, A.A., et al. Connectivity, and complex systems: learning from a multi-disciplinary perspective. Appl Netw Sci 3, 11 (2018). https://doi.org/10.1007/s41109-018-0067-2
Acknowledgment:
My Ph.D. project is a part of the Marie Skłodowska-Curie Actions Innovative Training Network ((MSCA ITN)) ‘I-CONN’ project. This project has received funding from the European Union's Horizon 2020 research and innovation programme under the Maria Skłodowska-Curie grant agreement No 859937.
References:
- Wainwright, J., Parsons, A. J., Schlesingerz, W. H. & Abrahams, A. D. Hydrology-vegetation interactions in areas of discontinuous flow on a semi-arid bajada, Southern New Mexico. J. Arid Environ. 51, 319–338 (2002).
- Reinhardt, L., Jerolmack, D., Cardinale, B. J., Vanacker, V. & Wright, J. Dynamic interactions of life and its landscape: feedbacks at the interface of geomorphology and ecology. Earth Surf. Process. Landforms 35, 78–101 (2010).
- Turnbull, L. et al. Connectivity and complex systems: learning from a multi-disciplinary perspective. Applied Network Science vol. 3 1–49 (2018).
- Barabási, AL. The network takeover. Nature Phys 8, 14–16 (2012).
- Turnbull, L., Wainwright, J., 2019. From structure to function: Understanding shrub encroachment in drylands using hydrological and sediment connectivity. Ecol. Indic. 98,608–618.
Shubham Tiwari is a Ph.D. student in the Department of Geography at Durham
University. He is also working as an Early-Stage Researcher in the ‘I-CONN’ project
funded by the European Union. His research focuses on land degradation feedbacks in
dryland ecosystems, and he uses complexity science to better understand them.
He graduated from IISER Bhopal with a BS-MS in Earth and Environmental Sciences in
2019. His Master’s Thesis work focused on the
emergence of rainfall patterns in Murray Darling Basin in Australia and the Ganga
River Basin in India, with results published in the Journal of Hydrology and Nature
Scientific Reports. When he is not compiling codes and reports, Shubham spends
most of his time, playing tennis and board games. He is also easily distracted and
enjoys multitasking failures.
You can get in touch with Shubham on Twitter (@EcogeoNetwork) or Instagram
(@shubhamrt_) .